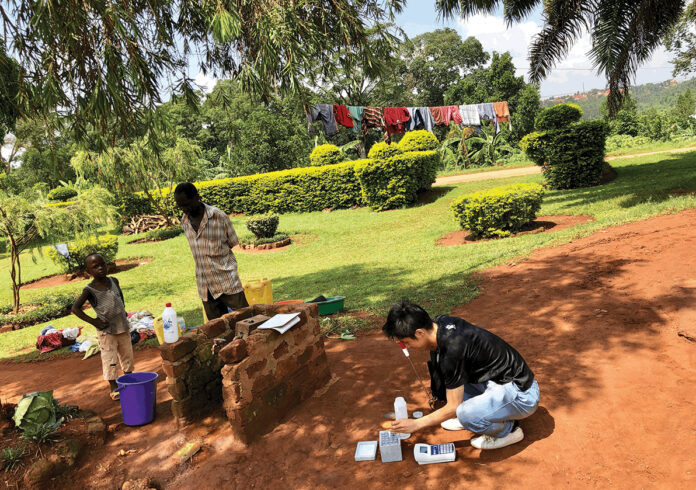
Intermittent operation of water supply networks is widespread, including in sub-Saharan Africa. Edo Abraham, Joost Verbart and Jan Peter van der Hoek look at the opportunities to use digital technologies to improve management.
Driven by the Millennium and, now, Sustainable Development Goals, many rapidly urbanising cities in sub-Saharan Africa have increased household access to improved water supply. Even so, universal access to ‘continuous supply’ of safe drinking water from piped water on premises still remains a distant goal. While many such cities – Kampala and Addis Ababa, for example – are making progress developing groundwater and surface water sources, and expanding piped water infrastructure, the need for ambitious extension of services to an even more rapidly growing urban population means intermittent water supply (IWS) will probably be the norm for cities for some time to come.
Intermittency exists partly because there is not enough supply capacity to pressurise the growing distribution system to supply all consumer taps at the same time, and partly because of the leaky nature of the existing infrastructure. Water loss levels of more than 30% from distribution pipes, combined with apparent losses (billing errors and theft), typically contribute to loss of 50% for many capitals in sub-Saharan Africa.
Although often considered suitable as a short-term coping mechanism, IWS as operated at present causes a vicious circle of multifaceted problems in the long term. First, water quality degrades during distribution, causing waterborne diseases. This is a result of: contaminant intrusion into the leaky pipe system under low or negative pressures; increased in-pipe deposits and related biochemical processes; unsanitary transport and storage by consumers; and residual chlorine depletion in storage (see Sakomoto, Abraham, Lutaaya, 2020). In addition, the large pressure variations that occur because of intermittent operation result in more bursts and weakening of the infrastructure. In turn, increased leakage and rehabilitation expenses deplete the already insufficient funds of utilities and reduce the accessibility of water for customers at the ends of the distribution system.
The coping costs for users resulting from intermittent supply are not equitable either. The poorest are disproportionately affected by the need to buy storage tanks, to treat or boil water, or by having to deal with waterborne diseases. Intermittency also exacerbates gender imbalances, as women and children share the brunt of the labour in water collection. Currently, more than a third of urban households in sub-Saharan Africa spend more than 30 minutes a day collecting water. The way that water is distributed in intermittent systems means there are spatial differences in impacts across a city. A customer’s location, the topology of the water system and associated physical constraints, and other spatial socio-economic differences will constrain whether intermittency can be scheduled equitably. The duration of intermittent supply also varies from city to city. In Kampala, for example, most connections are supplied for 24 hours every day and some areas are without water for a few hours a day, whereas Addis Ababa has areas that are supplied all the time and areas supplied for a few hours per week.
In recent years, smart water systems in many developed cities have leveraged advances in water quality sensors, pressure and flow loggers, and cloud-based analytics and IT solutions to monitor systems in real time, as well as increase efficiencies across the water cycle from source to tap. Some utilities have combined the discretisation of the network into district metered areas (DMAs), with advanced hydraulic modelling and real-time control of network pressure levels to reduce leakage. Software and sensing tools for leakage detection, and localisation for repair, water quality monitoring in the network, as well as intrusion detection, have been well studied and are currently offered by multiple consultancies and technology companies as products. Moving towards these smart water systems has proven to be beneficial for both utilities and customers. Likewise, these opportunities should be seen as desirable objectives for IWS systems (Figure 1).

Water quality in IWS systems
Although IWS is increasingly common in many developing cities, and will be so for some time, these mature smart technologies – made for continuous supply systems – are rarely adapted to solve operational and design problems for intermittent systems. For example, research is limited on IWS and its effects on water quality and, therefore, on public health. Although it is known that water quality deterioration in IWS mainly takes place in the distribution system, not much data exists that shows the impact on water quality and health quantitatively. There are also no clear guidelines on how a given intermittent system should be operated to safeguard quality at the customer side. By studying the mechanisms that result in poor water quality in distribution, we can mitigate these problems. Advances in rapid water quality sensors can be combined with hydraulic modelling to easily map water quality across a distribution system, identify pathways for quality degradation, and propose interventions for IWS systems (see box for details of work contained in Sakomoto, Abraham, Lutaaya, 2020).

Leakage control in IWS
Similarly, the repair of leaks is a priority for IWS, to reduce both economic losses and increase supply. To apply advanced leak reduction strategies, leak detection and localisation is key. After a utility has become aware of a leak in a certain area, its localisation can be further determined by various leak detection methods. This awareness is usually created by hydraulic monitoring, where a deviation from expected pressure levels and flows (i.e. a ‘baseline scenario’) could be attributed to a leak with a certain probability.
Modelling these baseline scenarios presents major challenges for IWS systems. For systems with continuous supply, pressure can be monitored constantly at various locations in the distribution system, and pumps can be operated with feedback to maintain sufficient pressure throughout the system. Furthermore, as the availability of water becomes reliable, users develop regular water consumption patterns. The constantly regulated pressure levels and reliable demand models, in combination with a range of smart meters and hydraulic models, allow utilities to model baseline scenarios. Leak diagnosis and localisation is then achieved through the analysis of anomalies measured by pressure and flow loggers.
Modelling this baseline scenario is a lot more complicated for IWS systems. This is because operating pressures vary daily and locally, as flow magnitudes, or even directions, change when different service areas are closed off or are connected to the large diameter mains transport system. This operational scheme of closing and connecting sections to the main supply system is often regulated by a local branch of the water utility. In some cities, whole service areas might suddenly get water if extra water is available. Although the intentions of this water provision are good, it introduces more irregularity to the supply pattern and adds complexity to leakage diagnosis processes.
Another major hurdle on the road to smart IWS is the lack of knowledge on accurate customer demand patterns, mainly as a result of the use of storage tanks in response to unreliable supply times. A customer water meter in an IWS system measures people filling their storage instead of end-use activities consuming water, which results in a very different demand pattern where ‘all taps are open all the time’. Adding the cultural difference in water use among different communities within the city, this results in a water consumption pattern that is often unknown and completely homogenised spatially. On top of this, utilities often face a lack of reliable GIS data on the water distribution system. The irregular operational scheme, lack of reliable GIS data, and unknown demand patterns are major barriers to overcome before algorithmic detection of leaks can be a success.
Despite these barriers, unique opportunities can be identified in IWS systems. Whereas it is challenging for utilities with continuous supply to construct DMAs in their often strongly meshed water distribution networks, closed off areas are created daily during IWS. By installing smart flow meters that wirelessly transmit measurements to a SCADA system, these sectorised intermittent supply zones could be easily converted to district metered areas (DMAs) that can be monitored in near real time. Furthermore, although the baseline scenario can vary significantly sometimes, a weekly baseline scenario could well be established by applying a consistent supply scheduling strategy. Leak awareness could then be created by comparing daily monitored measurements with the weekly baseline scenarios.
The way ahead
To manage IWS effectively, we need to develop new decision support tools that make it possible to optimise operational rules (when and where supplies should be directed, explicitly considering equity concerns) to manage pressure levels better for customers and, at the same time, mitigate leakage levels across the system. Resources that are required for the local utility to implement these include: up-to-date GIS models of water systems; consistent scheduling of supply; surveys to accurately represent the demand at customer taps; and smart metering at end-user and strategic locations. While sensing and digital technologies are essential for smart systems, their translation into water quality and water quantity improvements can only be realised through improved governance and management, and by building a skilled workforce within utilities (Simukonda et al, 2018). Then the capital investments required for implementing smart metering provide an interesting business case, from which a return on investment can be calculated based on the costs and savings for the local utility. While the installation of the smart meter networks requires advanced tools and expertise in hydraulic modelling and data-science, operating such smart systems in leak repair and water quality control would require limited technical and managerial skills.

These challenging conditions call for international guidelines for IWS systems that local utilities can use to manage their system efficiently and equitably. It is important to develop tailored approaches for IWS systems by using emerging technologies, to support local utilities in decision making and increase the water availability for transition to continuous supply, where possible. Together with local water utility partners, it is vital to study the interplay of existing intermittent system management, end-user behaviour, distributed storage and pumping, the level of leakage, microbiological water quality, and socioeconomic issues. This can build and strengthen local capacity in research in the process.
As water researchers, we contribute to solutions by collecting and assimilating water network data and constructing hydraulic models, building algorithms for optimal sensor locations to localise leaks in DMAs during continuous supply schedules, and supporting the creation of regular supply schedules, which could be coordinated with leakage diagnosis campaigns. Utilities then benefit from reduced costs, operational ease and improved services. By developing such decision support tools and implementing smart IWS systems, we can improve water quality and quantity, and increase safe drinking water access for many. •
More information:
Sakomoto, Takuya, Mahmood Lutaaya, and Edo Abraham. Managing water quality in intermittent supply systems: the case of Mukono Town, Uganda. Water 12, no. 3 (2020): 806.
Kondwani Simukonda, Raziyeh Farmani and David Butler. Intermittent water supply systems: causal factors, problems and solution options. Urban Water Journal 15, no. 5 (2018): 488-500.
Dr Edo Abraham is Assistant Professor in Water Management and Control Systems Engineering, TU Delft, The Netherlands
Joost Verbart is MSc Research Student, TU Delft
Dr Jan Peter van der Hoek is Professor of Drinking Water Engineering (TU Delft), and Head of the Strategic Centre at Waternet
Water quality in intermittent water supply systems
The map (below) shows an example of an overlay of rapid mobile sampling of multiple chemical and microbial water quality parameters along an intermittent water distribution system (red) and past burst and leak locations (green) (from Sakamoto et al, 2020).
Through rapid water quality tests taking a few minutes (Photometer PF-12Plus and NANOCOLOR tube tests, and Compact Dry EC Nissui for coliform and E. coli), it was possible to show deterioration of free chlorine levels in the network and of microbial water quality (e.g. E. coli) after the leaky part of the network. This makes contaminant intrusion in low pressure times, as well as bacterial regrowth in pipes, potential culprits.
By simulating the water age and chlorine decay through the system during continuous supply days, it was possible to test and recommend booster chlorination at certain supply tanks that would guarantee sufficiently high residual chlorine levels at downstream supply points. However, further research is needed to test the efficacy of this intervention in the real system, especially during first flushes when service resumes after intermittency.
A more detailed water quality mapping across the same parts of the network, through a combination of more frequent repetitive grab sampling and remotely communicating in-pipe sensors, could give more insight into the dynamics of disinfectant residual concentrations and indicator bacteria as service changes. This would allow better understanding of the impact of different intermittent operations and, in turn, help control the impact on water quality.
